Posted on Wed, 09/18/2019 - 06:35
By David B. Layzell, Jessica Lof and Geoff Martin
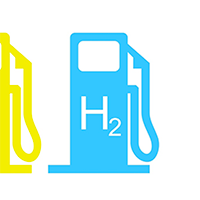
His large, heavy engines quickly displaced steam and found a place in ships and trains where size was not important3. However, it was not until the 1960s that technological improvements in design and materials allowed diesel-fueled vehicles to dominate the commercial trucking industry4. They have done so ever since.
Now, over 50 years later, there is a new kid on the block: electric drive vehicles that are vying to take the place of diesel in the heavy-duty vehicle marketplace. Air pollution and climate change concerns are major reasons for the change, but not the only reasons.
Figure 1. Front cover of the new Future of Freight (Part B) report from CESAR. Click on the image to download.
Electric-drive heavy-duty trucks come in two ‘flavours’: battery-electric (BE) such as those from Tesla5 or XOS6, and hydrogen fuel cell electric (HFCE) such as those from Nikola7 and Toyota8. Are they up to the challenge?
In a new report, released today (Figure 1), CESAR explores this question using techno-economic and environmental assessment (TEEA) tools. This blog post provides a synopsis of the report’s findings on the well-to-wheels flow of energy through four systems9 supporting a ‘Typical Shipment’ for heavy freight in Canada moving a 27 tonne gross load over a distance of 750 km. While most of our calculations have relevance to all Canada, on aspects where there are provincial differences, we took an Alberta perspective.
To illustrate each system, we use Sankey diagrams (Figure 2), where the height of each horizontal bar is proportional to the magnitude of energy flowing from its extraction through to the kinetic energy needed for a Typical Shipment (units of GJHHV/trip). We also report on the well-to-wheels greenhouse gas (GHG) emissions, system-level economics and logistical ‘fit-for-service’ in Alberta’s freight transportation sector.
The Fossil-Diesel, Internal Combustion Engine (FD-ICE) Energy System
Figure 2. Sankey diagrams showing the well-to-wheels flows of energy through four different systems capable of providing the 3.8 GJ of kinetic energy needed to move a 27 tonne heavy duty vehicle a distance of 750 km (i.e. a Typical shipment in Canada). Non percentage values are in units of GJ/trip. FD-ICE, Fossil diesel to internal combustion engine; G-BE, public grid to battery electric vehicle; NG-HFCE, Natural Gas to hydrogen fuel cell electric; WS-HFCE, Wind and solar to hydrogen fuel cell electric.
Energy Flows. This incumbent energy system consumes about 280 L (10.8 GJHHV) of diesel fuel for the ‘Typical Shipment’. Given a 35% engine efficiency for a heavy-duty diesel engine10, 3.8 GJ of kinetic energy is required per trip (Figure 2A, orange flow on right). We used this number as the kinetic energy requirement for the alternative electric drive energy systems.
To provide the energy for this trip, 14.4 GJ of energy inputs are required in the fossil diesel – internal combustion engine (FD-ICE) energy system (Figure 2A, left side), resulting in a well to wheels efficiency of 26% (kinetic energy out ÷ all energy in), with most of the losses occurring at the power train.
Emissions. Well-to-wheels GHG emissions of 1085 kg CO2e/trip are associated with the FD-ICE energy system (Figure 3). Therefore, to meet Canada’s 2050 climate change commitments, viable alternative energy systems will need to reduce GHGs by about 84% or to 178 kg CO2e/trip, reflecting an 80% reduction of 2005 level of freight diesel emissions. With the majority of emissions coming from the vehicles, an alternative, zero-emission fuel is required.
Costs. The FD-ICE system also sets a target for the cost of alternative fuels at $64/GJ of kinetic energy (Figure 4) applied to the wheels based on the pre-tax, retail cost of diesel. Of the $64, 46% can be attributed to the cost of the energy feedstock (crude oil), 45% to the conversion of the oil into diesel, and 8% for its distribution and retail.
The Grid to Battery Electric (G-BE) Energy System
Energy Flows. Battery electric HDV powertrains are considerably more efficient than the internal combustion engine of a diesel vehicle (68% as compared to 35%).
“…in the Alberta scenario modeled here, the well-to-wheels efficiency of the G-BE energy system is, at 34%, higher than the 26% conversion efficiency for the FD-ICE energy system.”
However, this comparison ignores the upstream efficiency associated with extracting the energy from nature and providing it as electricity or diesel in the retail location. As shown in Figure 2A and B, these upstream stages are more energy efficient in the FD-ICE (~74%) than in the grid to battery electric (G-BE, ~49%)11 energy system if deployed in Alberta. [In other Canadian provinces that have grids dominated by large hydropower resources, the upstream conversion efficiency for the G-BE energy system would very high.]
Overall, in the Alberta scenario modeled here, the well-to-wheels efficiency of the G-BE energy system is, at 34%, higher than the 26% conversion efficiency for the FD-ICE energy system.
The electricity demand needed to support a typical shipment is substantial. At 5.6 GJ (1,556 kWh) per trip, it is equivalent to that electricity used by 78 Alberta households for a day12.
Emissions. In Alberta, the G-BE energy system is not a climate change solution for freight transport. As shown in Figure 3, with the 2016 Alberta grid, the well-to-wheels GHG emissions would be higher than that of the FD-ICE energy system. Even if Alberta were to get off coal and use renewables to provide 30% of generation (i.e. 2030 Grid in Figure 3), the GHG emissions would still be 3X higher than the target of 178 kg CO2e/trip.
Of course, in Canadian provinces that have a low-carbon grid, the G-BE system could make a significant contribution to climate change targets in the freight sector.
Figure 3. Well-to-wheels greenhouse gas (GHG) emissions associated with four energy systems capable of supporting a ‘Typical Shipment’ of 27 t gross weight X 750 km. CCS, carbon capture and storage; See legend to Figure 2 for other abbreviations.
Costs. The G-BE energy system should be able to support a typical shipment at a fuel price that is equal to or less than the FD-ICE system (Figure 4). These calculations assume a significant cost premium to deliver the power at a rate that is needed to fuel a HD vehicle, so efficiency improvements in this area could make the G-BE energy system even more cost-effective.
Fit-for Service. A significant problem we identified with the G-BE energy system is that the weight of batteries required to support the ‘Typical Shipment’ would severely compromise the carrying capacity of the vehicle.
Even if these vehicles only carried sufficient batteries to travel 375 km before recharging, the refueling time with next-generation, high capacity chargers would be an unacceptable 1.5 hours, more than 20 times that for the FD-ICE system. While battery electric vehicles may work well for urban or local dray duties, the G-BE system faces significant challenges for long distance, heavy freight movement.
Future innovations in the energy density of batteries, the electrification of highways13, or in battery-swapping business models14, may be able to address these challenges.
Fuel Cell Electric Vehicles with Hydrogen from Natural Gas (NG-HFCE) or Wind & Solar (WS-HFCE)
Energy Flows. The hydrogen fuel cell electric (HFCE) heavy-duty vehicles are another option for an electric powertrain that has sparked the interest of the freight sector. Unlike the battery electric alternative, fuel cells on board the vehicles convert stored hydrogen gas into the electricity needed for the electric drive axles. A bank of batteries is also included to allow regenerative breaking and to provide additional thrust when accelerating or going uphill. In this hybrid-electric system, the fuel cells charge the batteries when their output exceeds the demand for moving the vehicle.
The powertrain of the HFCE option is less efficient than the battery electric alternative and the ‘Typical Shipment’ consumes 8.1 GJ/trip (57 kg) of hydrogen gas (Figure 2C and D) compared to an electricity demand of 5.6 GJ/trip for the G-BE energy system (Figure 2B).
Figure 4. Mid-point kinetic energy fuel cost estimates for fuels needed to support four energy systems. CCS, Carbon Capture and Storage; See legend to Figure 2 for other abbreviations.
In Alberta, hydrogen is commonly produced from natural gas using steam methane reforming to support the oil refining, petrochemical and fertilizer industries in the province. The carbon emissions are currently released to the atmosphere, but the process could be adapted to capture and permanently store the carbon in the province’s geological formations.
With additional purification and compression, and the capture and sequestration of 90% of the byproduct CO2, the resulting ‘blue hydrogen’15 production can supply the fuel for a HFCE freight transportation system (NG-HFCE). As shown in Figure 2C, this energy system would require 13.8 GJ/trip of natural gas (plus some renewable power to support electricity demand for gas compression) to move the vehicle with an overall well-to-wheels system efficiency of 27%, similar to the FD-ICE system.
Another option for low or zero-carbon hydrogen production is to use renewable power to split water into hydrogen and oxygen via electrolysis. Assuming the generation is at a wind or solar farm, 12.6 GJ of input energy would be required to produce sufficient ‘green hydrogen’ to support the ‘Typical Shipment’ (WS-HFCE), giving a well-to-wheels efficiency of 30% for the WS-HFCE energy system (Figure 2D).
Emissions. The GHG emissions of the NG-HFCE energy system are about 34% lower than that for the FD-ICE energy system, even if there is no effort to capture and sequester the CO2 that is produced (Figure 3). With carbon capture and sequestration (CCS), the well-to-wheels GHG emissions reduced by 79% compared to the FD-ICE energy system, coming close to meeting Canada’s commitments under the Paris climate change agreement. The WS-HFCE energy system has no GHG or air emissions.
“…the wholesale, per kilometer cost of producing a zero-emission transportation fuel (i.e. ‘blue’ hydrogen) is only 41% of the wholesale cost of producing diesel, a fossil fuel renowned for its contributions to air pollution and GHG emissions.”
Costs. For the WS-HFCE energy system, the cost of the renewable electricity, the high capital and operating cost for electrolysis and the challenges in moving the fuel to the fueling stations, positions this energy system as the most expensive of the alternatives considered here (Figure 4). As will be discussed in the next report in this series, there are other potential benefits of this energy system that could mitigate these higher costs.
In the NG-HFCE energy system, the feedstock (i.e. natural gas) fuel cost was the lowest at $2.83/GJ natural gas which converts to $8.19 per GJ of kinetic energy moving the vehicle (Figure 4). Therefore, the embedded cost of the primary fuel for the NG-HFCE energy system is less than one third the embedded cost of a barrel of oil in the FD-ICE energy system. Moreover, expressed as $/GJ kinetic energy, the cost of converting the NG to hydrogen is only 55% of the cost of converting crude oil into diesel (Figure 4). Consequently, the wholesale, per kilometer cost of producing a zero-emission transportation fuel (i.e. ‘blue’ hydrogen) is only 41% of the wholesale cost of producing diesel, a fossil fuel renowned for its contributions to air pollution and GHG emissions (Figure 4).
The problem with the NG-HFCE energy system is the cost of distribution and retail, estimated to be 12 times higher than that for the FD-ICE energy system (Figure 4). This can be attributed to our assumed use of tube trailers to move the hydrogen, and assumptions about having relatively small fueling stations with high capital and operating costs relative to the amount of fuel sold. While these assumptions are reasonable for actual fueling systems operating today, clearly the distribution and retail of hydrogen is a topic needing detailed analysis if Alberta is to work to build out a hydrogen economy in the province.
Fit for Service. The high torque, lower maintenance cost, fewer emissions and quieter operation of the electric drive heavy duty vehicles (i.e. G-BE, NG-HFCE, WS-HFCE) are highly compelling for the freight sector. Of these alternatives, the HFCE vehicles promise performance characteristics that are more similar to the incumbent FD-ICE in terms of vehicle weight and refueling times.
A major challenge is that the cost of these new electric drive vehicles are much more than the comparable ICE vehicles that currently dominate the market. This will only change with a shift in the vehicle market, and that is only likely to happen if lower fuel and operational costs help offset the higher capital cost for the vehicles.
Policies could help to make this happen, and such policies would benefit from the fact that many in the freight sector are keenly interested in the benefits of electric drive alternatives.
There is also a rising interest from companies that extract and refine fossil fuels. The fact that Alberta already has the technologies for making a zero-emission transportation fuel (i.e. ‘blue’ hydrogen) for 41% of the per kilometer cost of highly-emitting diesel fuel, has a number of companies in the province seeing a credible, compelling transition pathway to a low carbon future.
Next in the Future of Freight Series…
Given the importance of North American diesel demand to the oil-dependent Alberta economy, the next report in this Series explores the resource and economic implications for Alberta should any of the alternative energy systems become the dominant energy system supporting the heavy freight sector or other sectors that are currently reliant on diesel.
Acknowledgement
Having made this work possible, CESAR greatly appreciates the support of Alberta Innovates, Natural Resources Canada and the charitable foundations that have contributed to the launch of the Transition Accelerator, including the Edmonton Community Foundation, the Ivey Foundation and the Clean Economy Fund.
Footnotes
8 https://www.forbes.com/sites/sebastianblanco/2019/04/23/toyota-kenworth-expand-hydrogen-semi-truck-push-at-los-angeles-ports/#69ee8451d762
9 The full report actually explores five energy systems (including bio-based diesel), but for this blog we are highlighting the comparison of energy systems supporting electric drive vehicles with the incumbent FD-ICE energy system
11 This efficiency would be even lower if we modeled Alberta’s existing public electrical grid. Instead, we assuming a more efficient grid (projected for 2030) that contains no coal generation, and 30% of generation from renewables that are assumed to have 100% conversion efficiency.
12 The average Alberta household uses about 7200 kwh/year, or 20 kwh/day (https://energyrates.ca/residential-electricity-natural-gas/)
13 https://www.theguardian.com/environment/2018/apr/12/worlds-first-electrified-road-for-charging-vehicles-opens-in-sweden or https://www.theverge.com/2019/5/9/18538030/germany-ehighway-siemens-vw-group-electrified-cables-wires-overhead-electric-hybrid-trucks
15 ‘Grey’ hydrogen comes from reforming of fossil fuels without carbon management; ‘Blue’ hydrogen is also from fossil fuels, but the majority of the carbon emissions are not allowed to go to the atmosphere; ‘green’ hydrogen uses renewable power to electrolyze water and produce carbon-free hydrogen. See https://ifrf.net/ifrf-blog/hydrogen-securing-supplies-for-a-colourful-future/